Abstract
Background
Thyroid cancer is a leading endocrine malignancy, with anaplastic and medullary subtypes posing treatment challenges. Existing therapies have limited efficacy, highlighting a need for innovative approaches.
Methods
We analyzed 658 articles and 87 eligible clinical trials using bibliometric tools and database searches, including annual publication and citation trends, were executed using Web of Science, CiteSpace, and VOS Viewer.
Results
Post-2018, there is a surge in thyroid cancer immunotherapy research, primarily from China and the University of Pisa. Of the 87 trials, 32 were Phase I and 55 were Phase II, mostly exploring combination therapies involving immune checkpoint inhibitors.
Conclusion
The study’s dual approach verifies the swift advancement of thyroid cancer immunotherapy from diverse perspectives. Immune checkpoint inhibitors have become the preferred regimen for advanced MTC and ATC in late therapeutic lines. However, since ICB plays a pivotal role in ATC, current clinical trial data show that ATC patients account for more and the curative effect is more accurate. Anticipated future developments are inclined toward combination regimens integrating immunotherapy with chemotherapy or targeted therapies. Emerging approaches, such as bispecific antibodies, cytokine-based therapies, and adoptive cell therapies like CAR-T and TCR-T, are exhibiting considerable potential. Upcoming research is expected to concentrate on refining the tumor immune milieu and discovering novel biomarkers germane to immunotherapeutic interventions.
1 Introduction
Thyroid cancer, a predominant malignancy of the endocrine system, accounts for approximately 1% of all neoplasms. According to the World Health Organization’s 2022 classification of thyroid tumors, thyroid cancer is categorized into eight distinct groups: developmental abnormalities, follicular cell-derived neoplasms, thyroid C-cell-derived carcinoma, mixed medullary and follicular cell-derived carcinomas, salivary gland-type carcinomas of the thyroid, thyroid tumors of uncertain histogenesis, thymic tumors within the thyroid and embryonal thyroid neoplasms [1]. Furthermore, within the group of follicular cell-derived neoplasms, there are seven specific subtypes, each with its unique characteristics. These subtypes include: follicular thyroid carcinoma (FTC), invasive encapsulated follicular variant papillary thyroid carcinoma (IEFVPTC), papillary thyroid carcinoma (PTC), oncocytic carcinoma of the thyroid (OCA), poorly differentiated thyroid carcinoma (PDTC), differentiated high-grade thyroid carcinoma (DHGTC), and anaplastic follicular cell-derived thyroid carcinoma (ATC) [1]. The disease notably targets a younger demographic, specifically individuals aged 16–33, and exhibits a higher incidence among females [2]. Key risk factors include a family history of thyroid cancer, Hashimoto’s thyroiditis, prolonged exposure to ionizing radiation, excessive iodine intake, and obesity [3,4,5]. Global incidence rates have shown an uptick, with approximately 568,000 new cases reported in 2020 [6].
Anaplastic thyroid carcinoma stands out as the most aggressive and lethal subtype, characterized by a median survival duration of approximately five months and a one-year overall survival rate of 20% [7, 8]. Traditional therapeutic regimens encompass chemotherapy and radiotherapy, either as monotherapies or in conjunction with surgical intervention. Advances in our molecular understanding have led to the identification of BRAF V600E mutations in about 40% of cases, offering novel therapeutic opportunities through the combined application of BRAF inhibitors like dabrafenib and MEK inhibitors such as trametinib, which have demonstrated significant response rates in BRAF-mutated ATC [9].
Medullary thyroid carcinoma, an exceedingly rare neuroendocrine tumor originating from parafollicular cells, primarily relies on surgical intervention for disease management. Nonetheless, a subset of patients who are either unresectable or experience disease recurrence have limited options, often resorting to targeted or immunotherapeutic modalities [10, 11]. Multi-target tyrosine kinase inhibitors and selective anti-RET tyrosine kinase inhibitors have emerged as efficacious treatments, significantly improving clinical outcomes for these patients [12]. Multiple drugs inhibiting signaling or oncogenic kinases, including those targeting Platelet-Derived Growth Factor Receptor and Vascular Endothelial Growth Factor Receptor, have gained regulatory approval [13].
Despite these advances, the search for additional effective treatments for high-grade thyroid cancers remains an imperative. Immunotherapy has surfaced as a promising strategy, particularly for anaplastic thyroid carcinoma, with diverse approaches ranging from tumor-associated macrophage targeting, cancer vaccines, adoptive immunotherapy, monoclonal antibodies, to immune checkpoint blockade [14]. Notably, the FDA granted approval for the anti-PD-1 antibody pembrolizumab for thyroid cancer treatment in 2020, and clinical trials have substantiated the therapeutic efficacy of the anti-PD-1 antibody spartalizumab in locally advanced or metastatic ATC [11].
Employing bibliometric analysis, a methodology integrating mathematical and statistical approaches for comprehensive literature scrutiny, our study utilizes VOSViewer and CiteSpace software to examine publications on thyroid cancer immunotherapy from 1999 to 2022. We further incorporate an analysis of 86 clinical trials as the cornerstone of our investigation. Our findings affirm that immunotherapy for thyroid cancer is experiencing an acceleration in both the quantity and quality of research, with evolving focal points in the field. As such, a multi-dimensional examination of the current research landscape is warranted.
2 Materials and methods
2.1 Scientometric analysis
A database was built to search related literature for bibliometric studies via the Science Citation Index Expanded in Web of Science Core Collection (WoSCC). Medical Subject Headings (MeSH) database was utilized to obtain retrieval terms for the search strategy, TS = (“thyroid cancer” OR “papillary thyroid carcinoma” OR ” follicular thyroid cancer ” OR “medullary thyroid cancer” OR “anaplastic thyroid cancer” OR “papillary thyroid microcarcinoma”)AND TS = ((Immunotherapy) OR (PD-1) OR (PD-L1) OR (CTLA-4) OR (TIGIT) OR (LAG3) OR (TIM-3) OR (A2AR) OR (OX40) OR (ICOS) OR (4-1BB) OR (Cadonilimab) OR (AK-104) OR (Camrelizumab) OR (Nivolumab) OR (Pembrolizumab) OR (Sintilimab) OR (Atezolizumab) OR (Ipilimumab) OR (Durvalumab) OR (Avelumab) OR (Zalifrelimab) OR (Dostarlimab) OR (Balstilimab) OR (gen1042) OR (m7842) OR (SHR-1701) OR (Lifileucel) OR (Antibody Drug Conjugate) OR (Tisotumab vetodik) OR (TNF) OR (IFN) OR (Interleukin) OR (Adoptive Cell Therapy) OR (TIL) OR (TCR-T) OR (CAR-T) OR (CIK) OR (LAK) OR (DC) OR (Therapeutic Vaccine) OR (Therapeutic Vaccination)). The search was limited to English language articles and included articles and reviews published from 1999 to 2023. Finally, this search strategy retrieved 658 records up to January 19, 2023.
VOSViewer 1.6.18 and CiteSpace (6.1.R6) were used to visualize and analyze the data as bibliometric tools and convert the original data into visualization. Then we downloaded 658 results from WoSCC in the Plain Text File form and no duplicate record was found after processing, so there were still 658 records remained. Further, the composition of the database is as follows: there were 654 publications (99.39%) with abstracts, 658 publications (100.00%) with DOI number, 657 publications (99.85%) with subject categories, and 657 publications (99.85%) with cited references. The time range for the analysis was from January 1999 to December 2022, and top 50 levels from each time slice were selected for analysis.
In this study, we analyzed references, and keywords (timeline view analysis and burst analysis) through CiteSpace (6.1.R6). Meanwhile, we used VOS viewer to analyze countries and institutions, keywords and authors of this field. In addition, we chose Google Chart Tools (v1.0) to make a world map, which shows the countries and regions that have made contributions to this topic.
2.2 Clinical trails
In this study, we chose two fundamental data source of clinical trials: ClinicalTrials.gov (https://www.clinicaltrials.gov) and WHO ICTRP (https://trialsearch.who.int). Our search strategy in these databases consisted of the following criteria: 1) Condition or Disease/Condition = (“thyroid cancer” OR “thyroid neoplasms” OR “papillary thyroid carcinoma “OR”follicular thyroid cancer “OR” medullary thyroid cancer” OR “anaplastic thyroid cancer” OR “papillary thyroid microcarcinoma”) Other terms/Innervation = (Immunotherapy OR PD-1 OR PD-L1 OR CTLA-4 OR TIGIT OR LAG3 OR TIM-3 OR A2AR OR OX40 OR ICOS OR 4-1BB OR Cadonilimab OR AK104 OR Camrelizumab OR Nivolumab OR Pembrolizumab OR Sintilimab OR Atezolizumab OR Ipilimumab OR Durvalumab OR Zalifrelimab OR Dostarlimab OR Balstilimab OR GEN1046 OR M7824 OR SHR-1701 OR Adoptive Cell Therapy OR TIL OR TCR-T OR CAR-T OR CIK OR LAK OR DC OR Lifileucel OR Antibody Drug Conjugate OR Tisotumab Vetodin OR TNF OR IFN OR Interleukin OR Therapeutic Vaccine OR Therapeutic Vaccination). In total, 97 clinical trials were identified in ClinicalTrials.gov and 48 clinical trials were found in ICTRP. After removing duplicates by Trial IDs, a total of 86 clinical trials were included in our analysis.
Discussion
5.1 Epidemiological status of thyroid cancer
The cornerstone of treatment for most patients diagnosed with well-differentiated thyroid cancer (WDTC), particularly those of low-risk categorization, remains surgical resection. Nonetheless, individuals exhibiting high-risk attributes often require a more nuanced therapeutic continuum, incorporating elements such as thyrotropin (TSH) suppression and radioiodine therapy (RAI) [32]. The advent of immunotherapy in recent years heralds a transformative epoch in the management of thyroid malignancies. Immunotherapeutic strategies fundamentally aim to modulate endogenous immune responses or employ targeted pharmaceutical agents with well-defined objectives for antineoplastic effects. Notably, immunotherapy is predominantly employed for subtypes resistant to conventional treatments.
Among the spectrum of thyroid malignancies, medullary thyroid cancer (MTC) constitutes a minority, representing approximately 3–5% of cases [32]. The therapeutic axiom for MTC involves the identification and subsequent targeting of actionable mutational events. Additionally, high tumor mutational burden serves as a selection criterion for immunotherapeutic intervention, while cases lacking such criteria generally default to standard chemotherapy. Thus, the penetration of immunotherapy within the realm of MTC remains restricted. Anaplastic thyroid carcinoma (ATC), although rare, is the most aggressive variant of thyroid cancer, characterized by a median survival duration ranging between 5 and 12 months and a one-year survival rate of 20–40% [33]. These grim prognostic metrics render immunotherapy a pivotal frontier for therapeutic exploration in ATC.
Our investigation, employing both scientometric analysis and review of clinical trials, substantiates the accelerated development of immunotherapeutic modalities in thyroid cancer. A detailed analysis of the implications of these findings follows.
5.2 Targeted therapy for MTC and ATC
As our comprehension of the genetic and molecular underpinnings of thyroid malignancies deepens, targeted therapies for specific genetic mutations have evolved concomitantly. For instance, BRAF-V600E serves as a diagnostic cornerstone across multiple pathological subtypes of thyroid cancer and correlates with the therapeutic effectiveness of BRAF inhibitors such as vemurafenib and dabrafenib [34, 35]. Medullary thyroid carcinoma (MTC) frequently harbors mutations in the proto-oncogene RET [36]. Agents targeting this gene, such as selpercatinib and pralsetinib, have shown promise for MTC patients harboring these specific mutations [37]. The NTRK gene family includes NTRK1, NTRK2 and NTRK3. NTRK gene fusion is a carcinogenic driver for various tumor types [38]. NTRK gene fusion is very common in some rare tumors, but also occurs in solid tumors, but the probability is very small, which includes thyroid cancer [39]. In response to such genetic abnormalities, new targeted drugs have been designed to treat related malignant tumors. Anaplastic lymphoma kinase (ALK) is found in a subtype of anaplastic large cell lymphoma. The types and number of ALK-positive tumors are increasing year by year, including anaplastic thyroid carcinoma, non-small cell carcinoma and so on [40]. Targeting ALK through RNA interference, monoclonal antibodies, small molecule inhibitors, has benefited patients in personalized cancer treatment [40, 41].
5.3 Chemotherapy for MTC and ATC
Chemotherapy remains a critical modality in the treatment of both MTC and ATC. Dacarbazine, a frequently utilized chemotherapeutic agent, has demonstrated efficacy in MTC and shows enhanced effectiveness when combined with adjunctive therapies such as radiotherapy or other chemotherapeutic agents like cyclophosphamide, vincristine, and 5-fluorouracil [42, 43]. Docetaxel is a chemotherapeutic drug that interferes with cell classification by interfering with microtubule networks. Docetaxel has been shown to play an active role in the treatment of ATC, and thyroid tumors are significantly inhibited after combined treatment with radiotherapy [44].
5.4 Microenvironment of thyroid cancer immunotherapy
The occurrence, growth and metastasis of tumors are closely related to the internal and external environment of tumor cells, including surrounding blood vessels, various cells, signal molecules and extracellular matrix. Under homeostatic conditions, immune surveillance mechanisms serve to maintain cellular equilibrium, including the elimination of nascent tumor cells through intricate immunological pathways. When cancer cells appear in the body, NK cells first kill them, and dendritic cells will extract new tumor antigens to T cells, and then produce corresponding antibodies through the cooperation of T cells and B cells [45]. Conversely, tumor cells often employ a myriad of strategies to evade immunological detection, including the recruitment of immunosuppressive cells and the modulation of their own immunogenicity [46]. Myeloid-derived suppressor cells (MDSC) are immature bone marrow cells, which are significantly elevated in patients with thyroid cancer and can exhibit strong immunosuppressive effects [47]. In the process of thyroid cancer cells escaping from immune surveillance, the presence of MDSC and M2 macrophages can help cancer cells avoid the killing of the immune system [48].
5.5 Immunotherapy markers for thyroid cancer
As tumor treatment enters the stage of precise immunotherapy, it is necessary to find biomarkers to guide the use of immunotherapy. Programmed cell death 1 (PD-L1) is an important immunosuppressive molecule that promotes tumor growth by inhibiting the activation of T cells [49]. It can inhibit the proliferation and activation of T cells by activating PD-1 and weakening the key dephosphorylation process in T cell receptor pathway, and can further change its metabolism and function, and even lead to the death of T cells [50]. Notably, the BRAF^V600E mutation has been shown to induce PD-L1 expression, making it a viable immunotherapeutic target in thyroid cancer [51]. Tumor mutation burden (TMB) serves as a quantitative measure of genetic mutations within tumors. The higher the value of TMB is, the better the effect of ICB treatment will be [52]. Whole exome sequencing showed that thyroid cancer had the third lowest TMB (between 0.1 and 1 mut / Mb) across 27 tumors [53]. In recent years, the research on TMB has gradually matured, and it has also become a potential marker and was approved by the FDA in 2020. Especially when combined with PD-L1, it has been shown to be a marker available in certain cancer types.
5.6 Immunotherapy
5.6.1 Immune checkpoint blockades
Immune checkpoint blockades have inaugurated a paradigm shift in cancer immunotherapy. These checkpoints serve as pivotal immunoregulatory elements, safeguarding against autoimmunity while maintaining immune homeostasis [37]. Currently, Programmed Death Receptor-1 (PD-1), Programmed Death Ligand-1 (PD-L1), and Cytotoxic T Lymphocyte-Associated Antigen 4 (CTLA-4) are the most extensively investigated checkpoints [30]. Beyond these canonical checkpoints, our study also encompasses LAG-3 and TIGIT (NCT05347212 and NCT05286801). Our analysis includes 57 clinical trials concerning immune checkpoint blockades, many of which employ combination therapies for thyroid cancer.
5.6.1.1 Single immune checkpoint blockade
Our investigation identified 12 clinical trials (13.95%) utilizing a singular immune checkpoint blockade and all of them were phase II clinical trials. These trials span from the earliest, initiated in 2015 (NCT02404441), to the most recent, initiated in February 2022 (NCT05119296). Of these, 11 trials opted for anti-PD-1 antibody as an immune checkpoint blockade, while one chose PD-L1 as therapeutic target (EUCTR2015-000269–30-DE). Three of them have already concluded (NCT02404441,NCT02688608,NCT03072160) and with spartalizumab and pembrolizumab as the selected agents. NCT02404441 included patients with advanced malignant tumors, including melanoma, non-small cell lung cancer, triple negative breast cancer and anaplastic thyroid cancer. NCT02688608 and NCT03072160 chose pembrolizumab in the treatment of metastatic or locally advanced ATC and recurrent or metastatic MTC. Preliminary safety evaluations have been completed for these inhibitors.
5.6.1.2 Multiple immune checkpoint blockades
Cytotoxic T-lymphocyte antigen 4 (CTLA-4) and programmed cell death-1 (PD-1) are the most commonly used immune checkpoint inhibitors [54]. In our cohort, 14 clinical trials (16.28%) employed a combination of two checkpoint inhibitors, either alone or in conjunction with other therapeutic modalities: four in synergy with targeted therapy, two with chemotherapy and one with adoptive cell therapy. These trials were primarily phase II studies, with three classified as phase I. Various combinations of checkpoints were explored, but the majority (9 trials) chose PD-1 in tandem with CTLA-4. PD-L1 and TIGIT (NCT05286801), PD-L1 and CTLA-4 (NCT03122496),PD-1 and PD-L1 (NCT04400474),double PD-1 (NCT05659186) were selected for the remaining four clinical trials.
5.6.1.3 Immune checkpoint blockades combined with tyrosine kinase inhibitor
In our study, there are 29 clinical trials using one or two ICB combined with targeted therapies and 15 (17.24%) of them used tyrosine kinase inhibitor (TKI), such as famitinib, lenvatinib, surufatinib, encorafenib and cabozantinib (Table 5). With the emergence of sorafenib which is the first TKI approved by the FDA in November 2013 and is used for the treatment of advanced metastatic DTC which is ineffective with RAI treatment [55], numerous of clinical trials accured. Among them, the combination of ICB and TKI, such as some fixed treatment modes such as Cabozantinib and Atezolizumab(PD-L1), Cabozantinib and Nivolumab(PD-1) and Ipilimumab(CTLA-4), Lenvatinib and Pembrolizumab(PD-1) are still feasible ideas for the treatment of thyroid cancer.Table 5 The clinical trials using ICB combined with tyrosine kinase inhibitor
Among these trials, Cabozantinib is the most widely used TKI (n = 6), followed by Lenvatinib (n = 4). In the realm of ICB, 13 selected anti-PD-1 drugs were utilized. Regarding the combination of these two therapies, Lenvatinib combined with Pembrolizumab emerges as the most frequently employed combination, and it consistently yields clear experimental results across all clinical trials. Therefore, we speculate that Lenvatinib combined with Pembrolizumab should be considered a more effective combination.
The ATLEP trial, registered under EUCTR2017-004570–34-DE and conducted by the Medical Center—University of Freiburg in Germany, is an integral part of our statistical analysis. As illustrated in Table 5, this trial falls under the category of a phase II clinical trial. Its primary objective is to assess the efficacy of the combined regimen of lenvatinib and pembrolizumab in patients diagnosed with poorly differentiated thyroid carcinoma and anaplastic thyroid cancer. As our research progresses, the synergistic effects of this combination therapy are becoming increasingly evident, leading to a rise in the objective response rate (ORR). This study serves to further underscore the unique advantages of utilizing lenvatinib and pembrolizumab in the treatment of thyroid cancer. Currently, this clinical trial is ongoing, with plans for an extensive evaluation of the safety and efficacy of immune checkpoint blockade (ICB) combined with tyrosine kinase inhibitor (TKI) treatment. We eagerly anticipate favorable experimental outcomes that will lend substantial support to the adoption of this combination therapy.
IBC combined with TKI is the most promising treatment, and related clinical trials account for more than half of the number of immune checkpoint combined with targeted therapy. Studies have shown that the expression of PD-L1 in ATC was higher than that in other subtypes [56]. In addition, BRAF V600E mutation may lead to higher PD-L1 mRNA expression. Therefore, PD-L1 expression is proposed as a possible biomarker for immunotherapy. Figure 9 shows the common combination types in thyroid cancer immunotherapy, anti-PD-1 immune checkpoint inhibitors and tyrosine kinase inhibitor (lenvatinib). On the one hand, ICB regulates the immune system and promotes its attack on tumor cells by attenuating the inhibition of T cells. On the other hand, lenvatinib slows down tumor growth by blocking VEGF or VEGFR pathways and reducing blood vessels. In addition, VEGF inhibition can also reverse the phenomenon of immunosuppression in the tumor microenvironment by inhibiting regulatory T cells, M2 macrophages and myeloid-derived suppressor cells (MDSC).
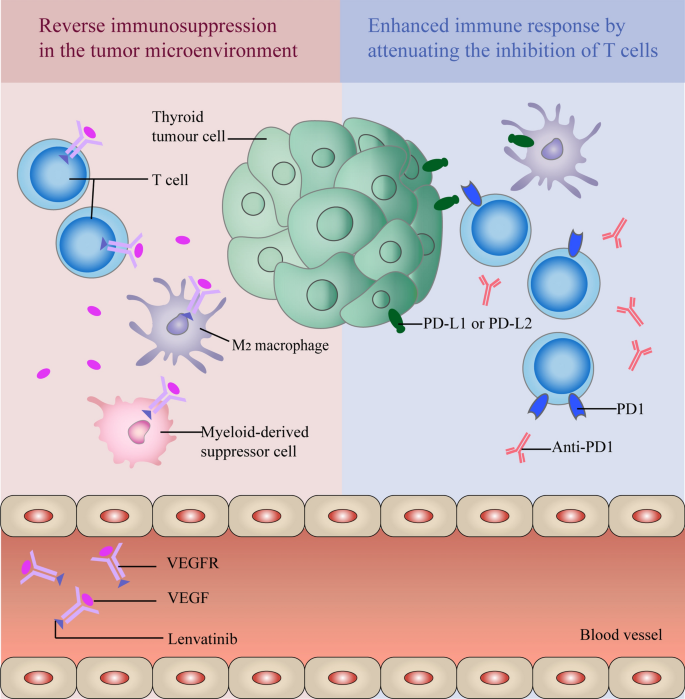
5.6.1.4 Immune checkpoint blockades combined with chemotherapy
Chemotherapy remains an essential pillar of cancer treatment. Together with surgery and radiotherapy, they are called the three major treatments for cancer. In our dataset,, there were 7 clinical trials (8.14%) combined with immune checkpoint blockades and chemotherapy. Because of the wide application of chemotherapy, most clinical trials have combined a variety of treatment methods, so 4 of the 7 clinical trials also combined with targeted therapy, one combined with radiotherapy. NCT03211117 was a phase II clinical trials using pembrolizumab, chemotherapy, and radiotherapy to treat patients with ATC. The clinical trials in our study show that docetaxel and doxorubicin hydrochloride are drugs which are usually used in chemotherapy. They can stop the tumor cell growth through a variety of ways, including cell killing, cell division inhibition, and metastasis prevention.
5.6.1.5 Immune checkpoint blockades combined with radiotherapy
Radiotherapy utilizes high-energy X-rays to shrink tumors and eradicate tumor cells [57]. In the treatment of thyroid cancer, radiotherapy is also a commonly used treatment method, such as iodine 131 treatment for differentiated thyroid cancer. In addition, radiotherapy can be more accurate by targeting molecules that are preferentially expressed on cancer cells. It is usually used when residual cancer cells still exist or cancer cells have spread to other sites after surgical removal of the thyroid gland [58]. Our study identified five clinical trials (5.81%) combining immune checkpoint inhibitors with radiotherapy (NCT03122496, NCT05659186, NCT04675710, NCT03215095, NCT03211117). These combinations, particularly the triad of pembrolizumab, chemotherapy, and radiation therapy, may offer enhanced therapeutic outcomes for patients with anaplastic thyroid cancer.
5.6.2 Adoptive cell therapy
Adoptive cell therapy (ACT) entails the isolation, amplification, and functional characterization of immunocompetent cells from patients with cancer, followed by their reinfusion into the patient to target neoplastic cells. Within the scope of our study, ACT is represented in 7 clinical trials: 4 exclusively utilize chimeric antigen receptor T-cell (CAR-T) therapies (4.65%), 1 combines CAR-T with chemotherapy (1.16%), and 2 engage tumor-infiltrating lymphocytes (TILs) alongside anti-PD-1 immune checkpoint blockade (ICB) (2.33%).
5.6.2.1 Tumor infiltrated lymphocyte (TIL)
TIL therapy is an emerging modality of cell-based immunotherapy that employs autologous immune cells harvested from the tumor microenvironment for therapeutic purposes [59]. Ontrasted with other immunotherapeutic strategies such as CAR-T and ICBs, TILs offer advantages in multi-receptor targeting, enhanced tumor tropism, and reduced adverse events. In our investigation, two clinical trials (2.33%) deployed TILs in combination with anti-PD-1 ICBs. Specifically, LN-145 and LN-145-S1 were used in conjunction with ipilimumab and nivolumab in a Phase II trial focusing on anaplastic thyroid cancer (NCT03449108). However, outcomes remain pending, necessitating further research.
5.6.2.2 Chimeric antigen receptor T-cell (CAR-T)
CAR-T therapy is an evolving form of immunotherapy that involves the genetic modification of T-cells to express specific chimeric antigen receptors (CARs), enabling targeted cytotoxicity against tumor cells [60]. Our study identified that ICAM1 (20%), GFRa4 (20%), and TSHR (60%) were the principal targets (NCT04420754, NCT04877613, ChiCTR1800014944, ChiCTR1800014936, ChiCTR1800017612). However, 4 of them are in phase I and only 1 is in phase I/II, indicating that CAR-T in the treatment of thyroid cancer is not mature enough so it still has a long way to go.
5.6.2.3 TCR-T, CAT-NK or NK or CIK
To enhance the efficiency of T-cell-mediated cancer cell eradication, T-cells are engineered in vitro to express tumor-specific T-cell receptors (TCR-T) [61]. TCR-T therapy is still in the process of development due to the high manufacturing cost and complex process, and the difficulty of improving T cell persistence [61, 62]. Compared with CAR-T therapy, CAR-NK has more advantages, such as higher safety and manufacturing feasibility [63]. Natural Killer (NK) cell therapy deviates from T-cell strategies by obviating the necessity for major histocompatibility complex (MHC) presentation, offering increased safety and control and also has higher heat in the current adoptive cell therapy [64]. Cytokine-induced killer (CIK) cells are another variant of T-cells, easily harvested and capable of MHC-unrestricted tumor cell lysis [65]. Therefore, whether they are used alone or in combination with other immunotherapy, they have broad prospects. Unfortunately, the above several adoptive cell therapies have not appeared in our clinical trials, but experiments and studies have shown that they are effective in the treatment of solid tumors. It is believed that there will be more clinical trials of these strategies for the treatment of thyroid cancer in the future.
5.6.2.4 Vaccine
Our study identified a total of 6 clinical trials (6.98%) that incorporate cancer vaccines; four were in Phase I and two in Phase II. Notably, the majority of these trials were initiated prior to 2015, with the exception of one (NCT03127098), which began in 2017 and combines a cancer vaccine with the cytokine IL-15. Vaccines for the treatment of cancer are mainly divided into peptide- and protein-based vaccines, cellular vaccines, genetic vaccines and other types of cancer vaccines [66]. NCT00004604 is a clinical trial of CEA RNA-pulsed DC cancer vaccine in the treatment of metastatic cancer, and it is also the earliest clinical trial in our study. Despite four decades of active research in this realm, the clinical translation of cancer vaccines remains limited. Continuous innovation, possibly in combination with other forms of immunotherapy, holds the promise of yielding more effective treatments [66]. It is believed that through continuous improvement tumor vaccines will achieve greater results.
5.6.3 Cytokine
Cytokines, as a class of small molecular proteins with multifarious biological activities, are synthesized and secreted by a variety of immune and non-immune cells. They serve dual roles within the tumor microenvironment, either fostering or inhibiting tumorigenesis [67]. Based on different functions, cytokines can be divided into interleukin (ILs), interferon (IFNs), colony-stimulating factors (CSFs), tumor necrosis factors(TNFs) and chemokines. Accordingly, cytokine-targeted immunotherapies can be divided into two overarching categories: cytokine-directed therapies and anti-cytotoxic factor therapies.
5.6.3.1 Monotherapy with Single Cytokine
Among the clinical trials exploring cytokine applications, 3 trials (3.49%) employed monotherapy using a single cytokine (NCT00098943, NTR7487, EUCTR2017-003028–59-NL). Specifically, Anakinra, a recombinant, non-glycosylated form of human interleukin-1 receptor antagonist, was chosen for treating anaplastic thyroid carcinoma in the latter two studies. NCT00098943 is a phase I clinical trial started in 2004, and CNGRC peptide-TNF alpha conjugate was selected. A number of studies have shown that interleukin is a cytokine that can affect the proliferation of thyroid cancer cells [67]. Particularly, IL-1α and IL-1β, members of the IL-1 family, have undergone extensive studies with respect to malignancies. IL-1α has been implicated in tumor-promoting activities, including tumor dedifferentiation and lymphangiogenesis [68, 69]. Conversely, while IL-1β has been shown to possess anti-tumor cell proliferative effects, its impact on ATC remains undefined [67]. Consequently, both trials in our dataset opted for anti-IL-1 therapies for ATC management.
5.6.3.2 Cytokine combination therapy
In our analysis, 5 clinical trials (5.81%) utilized cytokines in combination with other therapeutic modalities, such as immune checkpoint inhibitors, targeted therapies, chemotherapy, and radiation and vaccine therapies (NCT03127098, NCT04234113, NCT00004074, NCT02516774, NCT02390739). Compounds such as SO-C101, combined with immune checkpoint blockade pembrolizumab, and ALT-803, used in tandem with ETBX-011 vaccine, are associated with IL-15 activation. Furthermore, IL-12, IL-2, and TNF-α were employed in various phase I and II clinical trials.
5.7 The future of immunotherapy in thyroid cancer
While surgical resection remains the preferred treatment for resectable thyroid cancer, it is not without its challenges, such as high recurrence rates and potential damage to nerves in the neck, including the recurrent laryngeal nerve. Additionally, the use of iodine-131 in thyroid cancer treatment also carries associated risks, including gastrointestinal reactions, neck swelling, and parotid gland injury. However, the advent and continuous advancement of immunotherapies, particularly immune checkpoint inhibitors, have begun to alter the therapeutic landscape for various malignancies, including advanced and recurrent thyroid cancers. Immunotherapy, particularly immune checkpoint blockade, is at the center of this progress. Targeted therapies of thyroid cancer have been widely used in clinical practice. As discussed earlier, the combination of ICB and targeted drugs, particularly tyrosine kinase inhibitors, is a crucial direction for future development in thyroid cancer immunotherapy.
5.7.1 Strengths of the study
Our research presents a thorough analysis of the current state of immunotherapy in thyroid cancer, emphasizing the characteristics and emerging trends in this rapidly evolving field. We offer a robust reference for both clinicians and researchers, featuring meticulous, objective, and comprehensive insights. The statistical analysis of clinical trials constitutes a significant aspect of our research, and a comprehensive review of the strategies for immunotherapy of thyroid cancer based on statistical results. Finally, based on the above two points, we speculate the future development trajectory and research focus in this field.
5.7.2 Limitation of the Study
In our study, although we have conducted a comprehensive search for thyroid cancer immunotherapy as far as possible, the literature we collected may not be comprehensive due to the limitation of search strategy and the limitation of year and literature type. Moreover, the constraints of available visualization tools and statistical methods may not entirely represent the academic consensus. Finally, as far as clinical trials are concerned, it is a pity that the number of relevant clinical trials is small, and although there has been progress in thyroid cancer treatment with immunotherapy, our study shows that most of existing clinical trials are in phase I or II and many are still recruiting participants. Therefore, there is a need for a larger number of randomized, controlled phase III clinical trials to support these findings. Nevertheless, despite these limitations, our study provides invaluable insights that will inform future research in thyroid cancer immunotherapy.