In the United States, drug-resistant bacteria and fungi infect almost three million people per year and kill about 35,000. Antibiotics are essential and effective. However, in recent years overuse has led to some bacteria developing resistance to them. Now, researchers at Cold Spring Harbor Laboratory (CSHL) have created a new strategy against these drug-resistant superbugs.
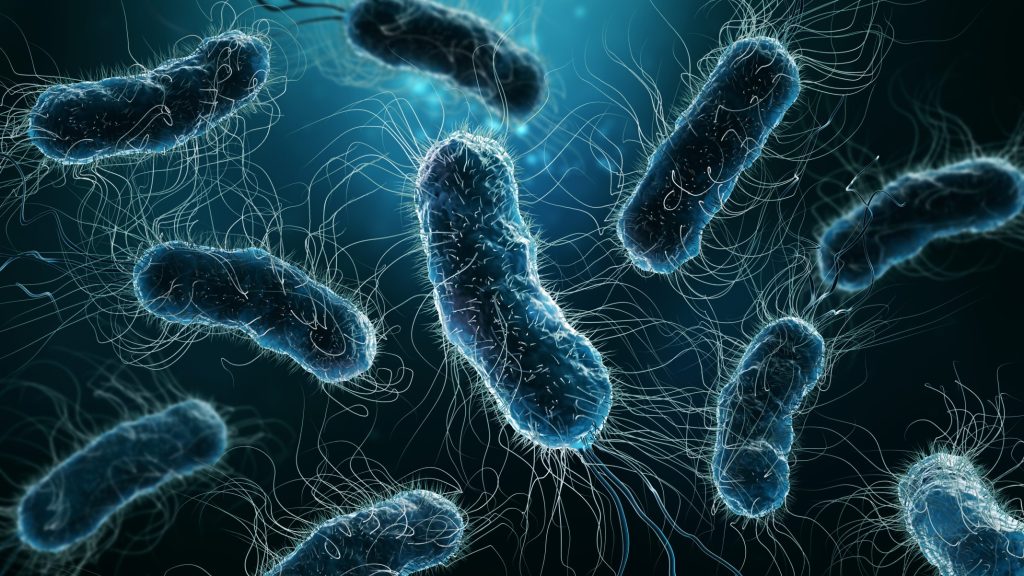
Their findings, published in Proceedings of the National Academy of Sciences, in an article titled, “Shapeshifting bullvalene-linked vancomycin dimers as effective antibiotics against multidrug-resistant gram-positive bacteria,” report of a shape-shifting antibiotic that may lead to new therapies against deadly infections.
“The alarming rise in superbugs that are resistant to drugs of last resort, including vancomycin-resistant enterococci and staphylococci, has become a significant global health hazard,” wrote the researchers. “Here, we report the click chemistry synthesis of an unprecedented class of shapeshifting vancomycin dimers (SVDs) that display potent activity against bacteria that are resistant to the parent drug, including the ESKAPE pathogens, vancomycin-resistant Enterococcus (VRE), methicillin-resistant Staphylococcus aureus (MRSA), as well as vancomycin-resistant S. aureus (VRSA).”
John E. Moses, PhD, professor at CSHL, who led the study, came up with the idea of shape-shifting antibiotics while observing tanks in military training exercises.
A few years later, Moses learned of a molecule called bullvalene. Bullvalene is a fluxional molecule, meaning its atoms can swap positions. Several bacteria, including MRSA, VRSA, and VRE, have developed resistance to a potent antibiotic called vancomycin, used to treat everything from skin infections to meningitis. Moses thought he could improve the drug’s bacteria-fighting performance by combining it with bullvalene.
Moses turned to click chemistry. “Click chemistry is great,” said Moses, who studied this revolutionary development under two-time Nobel laureate K. Barry Sharpless. “It gives you certainty and the best chance you’ve got of making complex things.”
Moses and his team tested the new drug in collaboration with Tatiana Soares da-Costa, PhD, group leader at the University of Adelaide. The researchers gave the drug to VRE-infected wax moth larvae, which are commonly used to test antibiotics. They found the shape-shifting antibiotic significantly more effective than vancomycin at clearing the deadly infection.
“If we can invent molecules that mean the difference between life and death,” he said, “that’d be the greatest achievement ever.”
The new findings pave a way for further studies into shape-shifting antibiotic drugs and overcoming the rapid emergence of multidrug-resistant bacteria and pathogens.