3D printing technology use cases have been somewhat lacking in the medical sector. Recently, a Ghent University spin-off project called Xpect-INX, started providing industry, clinicians, and researchers with unique materials (so-called ‘bio-inks’) to use for 3D printed cells, tissues, and possibly even entire organs.
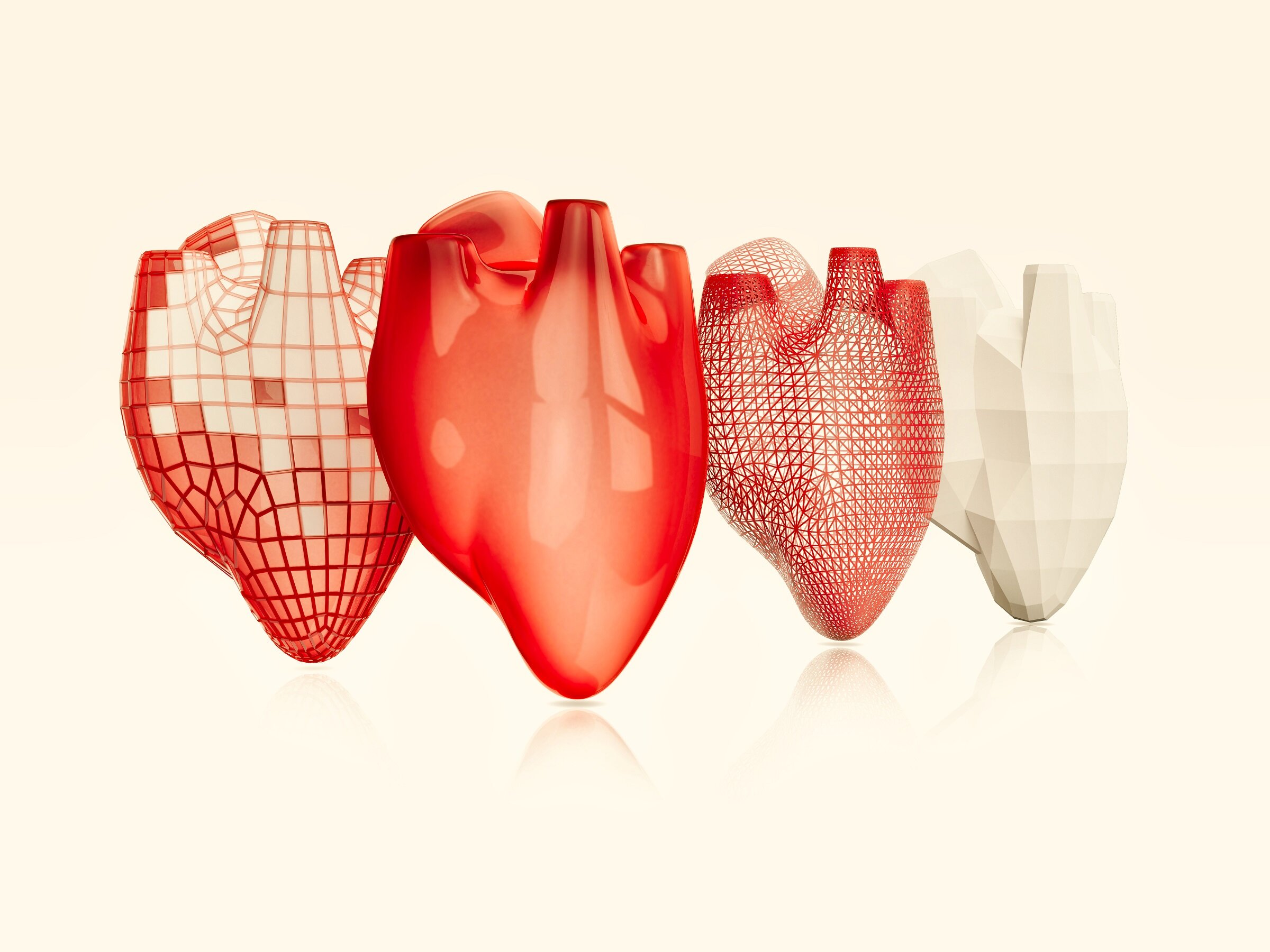
The Flanders region of Belgium has been a pioneer in the field of 3D printing technology for many years now, generating applications in space, fashion, and architecture. Sadly though, due to a lack of materials that can mimic the structure and function of biological tissues, the possibilities in the medical sector have been limited to 3D – printing of medical devices or anatomical models. However, with help from innovative materials developed at Ghent University, it is now possible to print human tissue.
In a Ghent University press release, Xpect-INX researcher and co-founder Jasper van Hoorick noted that 3D printing of cells and tissues is a very challenging process. 3D-printed cells must be able to grow and function like normal human cells in addition to being able to interact with existing cells until the body can fully integrate them. The key to this all is using appropriate and biocompatible materials, called “bio-inks.” Bio-inks are material mixtures that bear a strong resemblance to the natural cell environment. The bio-inks provide mechanical strength, a healthy growth environment for the cells, and they make it possible to process cells via various 3D printing techniques.

NEW OPPORTUNITIES
The potential applications of these ‘bio-inks’ are countless. They range from converting 2D cell cultures in Petri dishes to more realistic 3D cultures, repairing or replacing damaged cells or tissues due to illness or injury, and growing ‘mini organs-on-chip’ to screen for new medicines.
Currently, interventions such as breast reconstruction, replacement of a heart valve, or a meniscus often involve various complications. The combination of 3D printing with these bio-inks would offer a more sustainable and patient-specific solution. This way, damaged tissue can be replaced by new, body-specific tissue. The researchers expect that in the long term, even printing whole organs should be possible, which could overcome the shortage of donor organs.
Van Hoorick adds that this technology may provide opportunities to culture cells or mini-organs as a model for testing new drugs, substantially reducing the number of animal experiments.
SPECIFIC BIO-INKS FOR SPECIFIC APPLICATIONS
Every printer and application, from building an artificial cornea to the development of complex organs such as the liver, requires a specific, optimal ink. Currently, tissue biofabrication is still in its infancy, but it has come a long way. The Polymer Chemistry and Biomaterials research group, led by Peter Dubruel and Sandra van Vlierberghe, has been researching new polymers and bio-inks for these applications for more than ten years resulting in the Xpect-INX.
As stated, the potential use cases for these bio-inks are innumerably providing us with hope for the future. Stay tuned as we keep an eye on this technology.