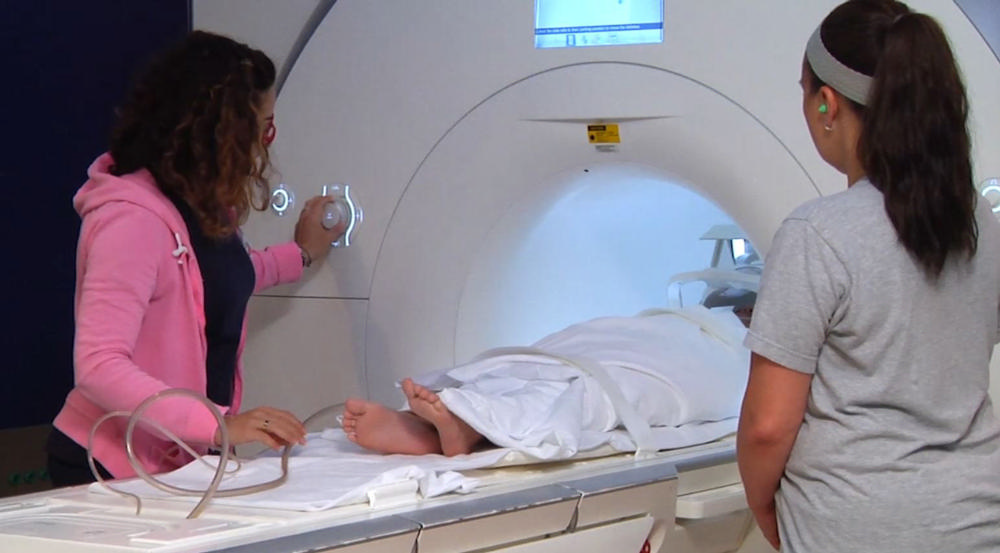
In unmedicated children with anxiety disorders, researchers at the National Institutes of Health have found overactivation in many brain regions, including the frontal and parietal lobes and the amygdala. They also showed that treatment with cognitive behavioral therapy (CBT) led to improvements in clinical symptoms and brain functioning. The findings illuminate the brain mechanisms underlying the acute effects of CBT to treat one of the most common mental disorders. The study, published in the American Journal of Psychiatry, was led by researchers at NIH’s National Institute of Mental Health (NIMH).
“The findings can help our understanding of how and for which children CBT works, a critical first step in personalizing anxiety care and improving clinical outcomes,” said senior author Melissa Brotman, PhD, Chief of the Neuroscience and Novel Therapeutics Unit in the NIMH Intramural Research Program.
Sixty-nine unmedicated children diagnosed with an anxiety disorder underwent 12 weeks of CBT following an established protocol. CBT, which involves changing dysfunctional thoughts and behaviors through gradual exposure to anxiety-provoking stimuli, is the current gold standard for treating anxiety disorders in children.
The researchers used clinician-rated measures to examine the change in children’s anxiety symptoms and clinical functioning from pre- to post-treatment. They also used task-based fMRI to look at whole-brain changes before and after treatment and compare those to brain activity in 62 similarly aged children without anxiety.
Children with anxiety showed greater activity in many brain regions, including cortical areas in the frontal and parietal lobes, which are important for cognitive and regulatory functions, such as attention and emotion regulation. The researchers also observed elevated activity in deeper limbic areas like the amygdala, which are essential for generating strong emotions, such as anxiety and fear.
Following three months of CBT treatment, children with anxiety showed a clinically significant decrease in anxiety symptoms and improved functioning. Increased activation seen before treatment in many frontal and parietal brain regions also improved after CBT, declining to levels equal to or lower than those of non-anxious children. According to the researchers, the reduced activation in these brain areas may reflect more efficient engagement of cognitive control networks following CBT.
However, eight brain regions, including the right amygdala, continued to show higher activity in anxious compared to non-anxious children after treatment. This persistent pattern of enhanced activation suggests some brain regions, particularly limbic areas that modulate responses to anxiety-provoking stimuli, may be less responsive to the acute effects of CBT. Changing activity in these regions may require a longer duration of CBT, additional forms of treatment, or directly targeting subcortical brain areas.
“Understanding the brain circuitry underpinning feelings of severe anxiety and determining which circuits normalize and which do not as anxiety symptoms improve with CBT is critical for advancing treatment and making it more effective for all children,” said first author Simone Haller, PhD, Director of Research and Analytics in the NIMH Neuroscience and Novel Therapeutics Unit.
In this study, all children with anxiety received CBT. For comparison purposes, the researchers also measured brain activity in a separate sample of 87 youth who were at high risk for anxiety based on their infant temperament (for example, showing a high sensitivity to new situations). Because these children were not diagnosed with an anxiety disorder, they had not received CBT treatment. Their brain scans were taken at 10 and 13 years.
In adolescents at temperamental risk for anxiety, higher brain activity was related to increased anxiety symptoms over time and matched the brain activity seen in children diagnosed with an anxiety disorder before treatment. This provides preliminary evidence that the brain changes in children with anxiety were driven by CBT and that they may offer a reliable neural marker of anxiety treatment.
Anxiety disorders are common in children and can cause them significant distress in social and academic situations. They are also chronic, with a strong link into adulthood when they become harder to treat. Despite the effectiveness of CBT, many children continue to show anxiety symptoms after treatment. Enhancing the therapy to treat anxiety more effectively during childhood can have short- and long-term benefits and prevent more serious problems later in life.
This study provide evidence—in a large group of unmedicated youth with anxiety disorders—of altered brain circuitry underlying treatment effects of CBT. The findings could, in time, be used to enhance treatment outcomes by targeting brain circuits linked to clinical improvement. This is particularly important for the subset of children who did not significantly improve after short-term CBT.
“The next step for this research is to understand which children are most likely to respond. Are there factors we can assess before treatment begins to make the most informed decisions about who should get which treatment and when? Answering these questions would further translate our research findings into clinical practice,” said Brotman.