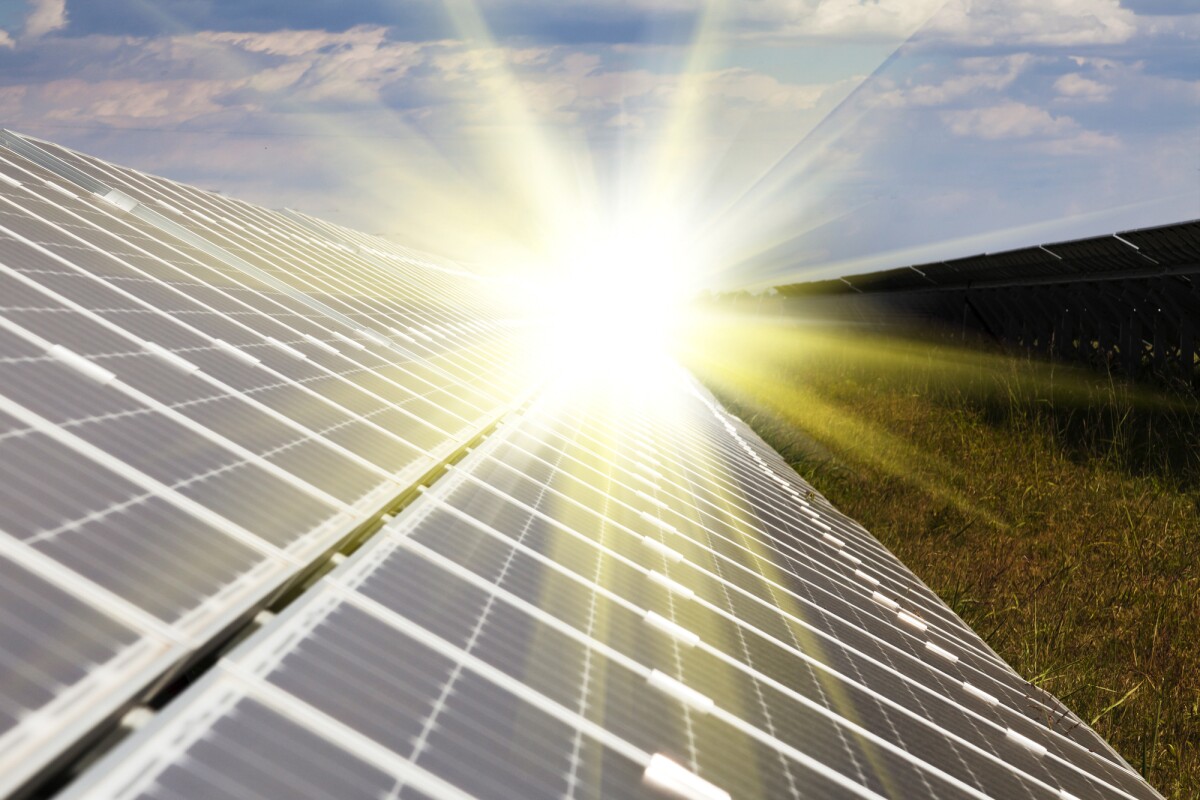
Solar cells made with quantum dots have achieved a new record efficiency, and been made more stable at the same time
One of the most promising, emerging solar cell technologies has received a major efficiency boost. Engineers at UNIST in South Korea have created quantum dot solar cells with a world record efficiency of 18.1%.
Quantum dots are essentially just tiny, circular semiconductor crystals that are incredibly efficient at absorbing and emitting light. The color of light they interact with can be set by changing their size, which makes them useful in display technologies or as sensors.
But where they might end up being most useful is in solar cells. Most commercial solar cells are made with bulk materials as the light-collecting layer, which means the whole surface absorbs the same wavelengths. But with quantum dots you can have multiple sizes that focus on a different part of the spectrum, boosting potential efficiency. As an added bonus, they’re cheap and easy to manufacture, and can even be made into a sprayable solution.
For the new study, researchers at UNIST tweaked the recipe a bit to improve the technology. Quantum dot solar cells made with organic materials have the highest theoretical efficiency, but unfortunately they suffer from defects that make them less stable in sunlight and weather – not ideal for devices designed to be out in the sun all day. To get around that, these solar cells are usually made with inorganic materials instead, but this limits their efficiency, the team says.
The UNIST team made their quantum dots out of an organic perovskite, and developed a new method for anchoring them to a substrate that allowed the dots to be placed closer together. This boosted the efficiency to a record-setting high of 18.1%, up from 16.6% in 2020. This record has been independently recognized by the National Renewable Energy Laboratory (NREL), which keeps an ongoing chart comparing the efficiency of different technologies.
Even better, the new solar cells were far more stable. They maintained their efficiency for 1,200 hours under normal conditions, and 300 hours at an elevated temperature of 80 °C (176 °F). They performed just as well after two years in storage.
Quantum dot solar cells still have a long way to go to catch up to the everyday silicon solar cells, the latter has had a half-century head-start and is rapidly approaching its theoretical maximum efficiency. Meanwhile, quantum dots have only really been in the lab since about 2010, when they had an efficiency of under 4%. Along with the efficiency gains, the inexpensive and simple manufacturing should help scale up the tech and make a wider range of surfaces photovoltaic.