Theoretical physicist saw his eponymous particle discovered after 48 years
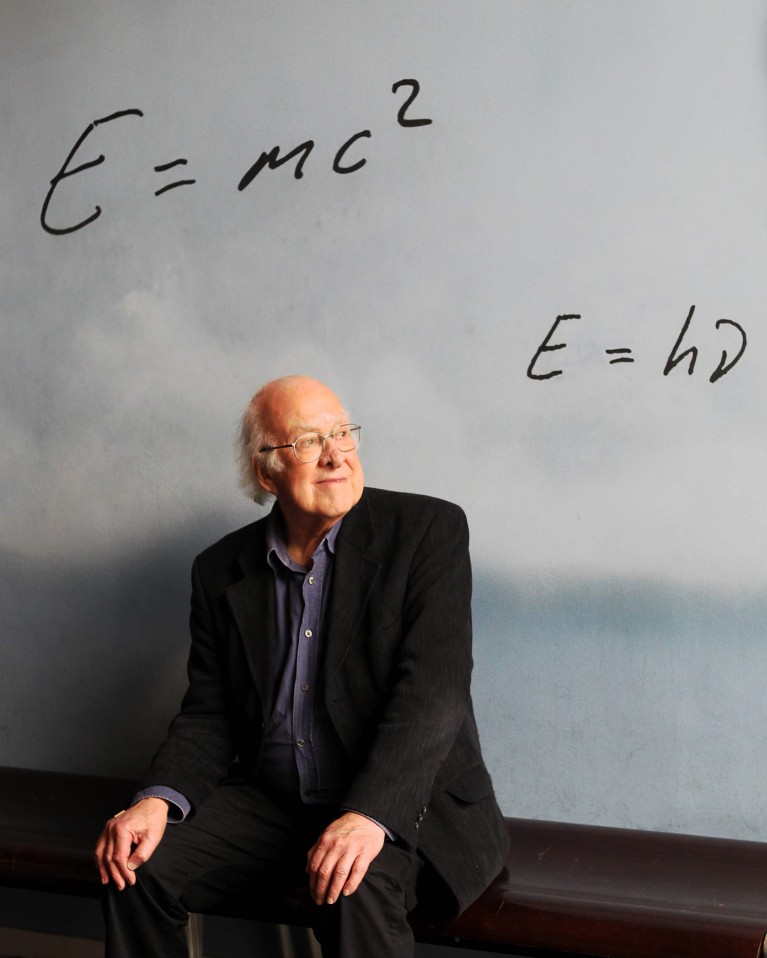
During a few weeks in the summer of 1964, Peter Higgs, a theoretical physicist at the University of Edinburgh, UK, wrote two short papers outlining his ideas for a mechanism that could give mass to fundamental particles, the building blocks of the Universe. His aim was to rescue a theory that was mathematically appealing but ultimately unrealistic because the particles it described had no mass. The second paper drew attention to a measurable consequence of his proposal — it predicted the existence of a new massive particle. Nearly half a century later, the discovery of the predicted particle brought Higgs, who has died aged 94, a share of the 2013 Nobel Prize in Physics.
The mechanism Higgs described became a key component of the standard model of particle physics during the 1970s, but the associated particle remained stubbornly elusive. Then, in 2012, two giant experiments run by more than 6,000 physicists at CERN, Europe’s high-energy physics laboratory near Geneva, Switzerland, discovered something with the appropriate properties. By then, the particle had achieved fame as the Higgs boson, although the self-effacing Higgs would usually refer to it as ‘the scalar boson’ in reference to its key characteristic of having no intrinsic spin.The ‘brazen’ science that paved the way for the Higgs boson (and a lot more)
Higgs was born in Newcastle upon Tyne in 1929, but his father’s work as a sound engineer for the BBC took the family to Bristol, where Higgs attended Cotham Grammar School. There, he spotted several mentions on the honours boards of a previous pupil, Paul Dirac, who had earned a share of the 1933 Nobel Prize in Physics for his work in quantum mechanics. Inspired, Higgs took up physics at King’s College London, where he obtained a PhD in 1954.
As a hitch-hiking student, Higgs had discovered a liking for Edinburgh, so in 1960 he was happy to be appointed as a lecturer there. He picked up on a long-standing interest in symmetry in subatomic particle physics, inspired in particular by the work of the future Nobel prizewinner Yoichiro Nambu, a Japanese American physicist then at the University of Chicago in Illinois. In physics, symmetry is linked to the conservation of quantities such as energy, momentum and electric charge. Working on a theory that had an underlying symmetry but in which particles had no mass, Nambu was attempting to generate mass through a mechanism known as spontaneous symmetry breaking. However, such symmetry breaking would also produce massless particles with zero spin, for which there was no evidence.Peter Higgs: the man behind the God particle
This seemed a dead end, but in 1964, Higgs realized that it was possible to get round the difficulty using gauge theory, which has the kind of symmetry found, for example, in the established theory of electromagnetism. Higgs showed that the massless particles associated with spontaneous symmetry breaking become ‘absorbed’ into massive particles. He published two short papers on this theme1,2, the second of which explicitly predicted a massive spin-zero particle. It fell to other physicists to realize that this mechanism for spontaneous symmetry breaking was key to a mathematically coherent gauge theory of particle physics that unites the electromagnetic interactions between particles with the weak interactions involved in certain forms of radioactivity. This Nobel-prizewinning ‘electro-weak theory’ became one of the twin pillars of the standard model of particle physics, and was well established by experiments by the 1990s.
The field had seemed something of a scientific backwater in the 1960s, but Higgs was not working alone. Two other papers were published in 1964 on the mechanism3,4, one appearing just before his own. But only Higgs drew attention to the associated massive spin‑zero particle, which in the 1970s began to be called the Higgs boson. The catchy name stuck.
Around this time, after his marriage broke down, Higgs found he was losing his way in theoretical particle physics. In addition to teaching, he became involved in the union side of university life and wrote few physics papers. Nevertheless, as appreciation of his work on spontaneous symmetry breaking grew, he was increasingly asked to give talks, a popular title being ‘My life as a boson’. He retired in 1996.The Higgs boson turns ten
The award of the Nobel prize to Higgs and Belgian physicist François Englert — the surviving author of the paper published just before Higgs’s in 1964 — came as little surprise to anyone, including Higgs, because it had been mooted since the 1980s. However, interest in the Higgs boson had increased over the years, not only among particle physicists but also among the general public and the media. It reached fever pitch after the construction of CERN’s Large Hadron Collider, which was billed by many as the machine that would discover the last missing piece of the standard model. This intense interest brought fame at a level no one could have imagined in the 1960s, and the quiet physicist became a media star, before retiring for a second time when he reached 85.
A keen music lover, Higgs had little interest in the trappings of modern technology — he famously had no television and did not use the Internet. Yet he was far from being remote from the world. He had a strong social conscience and was a member of the Campaign for Nuclear Disarmament and Greenpeace at various times. Humble in many respects, with an infectious sense of humour, he was proud of the work that he had always known was important and which ultimately brought him fame.
Nature